An R&D project at a California facility was executed under commercial-scale operation to evaluate the “+ASPw/BFC” technology’s operational feasibility and air emissions control performance.
T. Cordova, J. Goodwin, T. Card and C.E. Schmidt
BioCycle March/April 2015, Vol. 56, No. 3, p. 49
An increasing number of facilities are employing innovative control strategies and technologies to minimize air emissions and energy use at their composting operations (Horowitz et al., 2013). The goal is to ultimately support the sustainability of the composting operations with regard to: Minimizing off site odor and air emission impacts; Meeting regulatory air emission requirements; and Reducing fuel and power consumption.
One of the most promising new composting technologies is the use of positively aerated static piles covered with a biofilter layer of finished compost. This technology is identified by the acronym, +ASPw/BFC. The performance of this technology was first quantified by the San Joaquin Valley Air Pollution Control District (SJVAPCD) Technical Assistance Program (TAP) project. The TAP project evaluated this technology on green waste composting using a pilot sized process and showed dramatic reductions in both energy usage and air emissions. These air emission reductions occurred with total nonmethane nonethane organic carbon (TNMNEOC) or relevant volatile organic compounds (VOCs), ammonia (NH3), and greenhouse gases (GHG) including carbon dioxide (CO2), methane (CH4), and carbon dioxide equivalents (CO2e) (Table 1).
However, two big questions left by the TAP project included:
1. Would it work at a commercial scale?, and
2. Would it work for other composting feedstocks?
Full-Scale Research Trial
During the fall and summer of 2014, Synagro, a national biosolids management company, decided to find out the answers to these questions at its South Kern Industrial Center (SKIC) facility located in Bakersfield, California. A full-scale research and development program was undertaken to determine the viability of changing its existing biosolids composting process from a negative aerated static pile discharging to an organic media biofilter, to a +ASPw/BFC.
This project had two separate phases. During the first phase (completed in July and August, 2014), eight separate full-scale piles were built and tested for a full compost cycle, which is the operating model of the site. Based on knowledge gained from the first phase, a second phase was completed in November 2014. The second phase allowed for further optimization of the composting strategy since the operating parameters of pile management, ASP watering strategy and positive air flow rate/pile are largely gleaned by “trial and error” and specific to site conditions and feedstock.
The R&D project was executed under commercial operation so that the demonstration would include all site challenges. These challenges included not only analytical performance data but also information regarding operational feasibility. The site zones used for the testing were isolated from the negative aeration system and outfitted with positive aeration. The biosolids mix was placed in the selected zones and then covered with finished compost. The zones were fit with an irrigation system. The compost was left in place for the full cycle, which concluded with pile break-down, inspection, sampling, and compost quality testing.
Air Emissions Testing
All sampling was completed in July, August and November 2014. It was conducted using the USEPA validated Surface Isolation Emission Flux Chamber modified as per the South Coast Air Quality Management District (SCAQMD) Rule 1133 for assessing emissions from advective flow compost sources. VOCs were analyzed per the SCAQMD Method 25.3; TNMNEOC, GHGs and ammonia were analyzed per SCAQMD Method 207.1. Figure 1 shows an example test location. A total of 77 test locations were analyzed for this project.
For each test, four locations were sampled on the top of each half cell. These test locations were equally spaced on a diagonal across the top of the half cell. From the test data, an emissions profile from a hypothetical test cell was built. Testing of a zone included selecting four flux chamber test locations on a diagonal transect from one corner of the zone to the other on equally spaced intervals (around 20 foot spacing). The resulting flux data that were used in concert with operational data (measured advective flow and composting metric data) to generate emissions data.
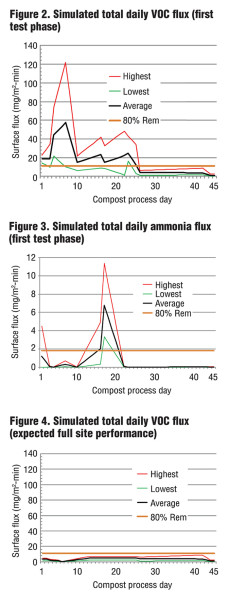
Figure 2. Simulated total daily VOC flux (first test phase)
Figure 3. Simulated total daily ammonia flux (first test phase)
Figure 4. Simulated total daily VOC flux (expected full site performance)
Figure 2 presents a simulated daily VOC emissions profile (in emission flux units, mg/m2 min) for the first phase of R&D. The calculated emissions profile consists of the measured data for the specific test days, with the emissions for the unmeasured days interpolated between the measured data points. The data is shown as a range from highest to lowest data points. Figure 3 presents the ammonia emissions data from the same period. This was done to provide insight as to the expected variability. In addition, the average of the four data points is shown. The horizontal line on the graphs represents the 80 percent control level based on the average measured uncontrolled emissions for the existing negative ASP discharging into a biofilter on all compliance tests over the life of the facility.
The ammonia emissions performance was outstanding during the first phase. However, in order to maximize site capacity, the VOC emissions performance needed to be improved. Using the information gained from the first phase, a second phase was initiated that included operating improvements related to pile and cover construction and moisture monitoring/control. The operational modifications did dramatically improve VOC performance. Figure 4 shows the expected full site VOC performance for this technology. Table 2 shows the VOC removal performance for this new technology, which was right in line with the TAP pilot results.
All field and analytical data were evaluated using the quality assurance/quality control (QA/QC) criteria as reported in the Protocol guidance document. All analytical methods were found to demonstrate acceptable performance, and these data can be used without qualification of data use or restriction without exception.
Table 3 shows the metrics of the ammonia removal performance for this technology. These emissions were comfortably within the site performance requirements.
Operational Parameters — Lessons Learned
Cover Thickness
This is obviously very important. Many of the problematic data points were discovered to have less than desirable cover. The recommended approach to managing cover thickness is to level the ASP to the extent possible and raise the entire surface with the cover material so that cover thickness can easily be visually determined.
Positive Air Flow Rate
Meeting the oxygen demand of the compost oxidation process while also using air flow to modulate the core temperature in the pile is quite the operator challenge. Monitoring compost temperature and oxygen levels plays into the strategy of operating the +ASP/wBFC and is key in this process.
Cover Moisture
Cover moisture is very important as well. Cover moisture can be observed visually; wetted finish compost looks much different than dry finish compost, so daily visual inspection is one part of the monitoring needs to assure acceptable conditions. In addition to the visual determination of cover moisture, an analysis of surface temperature data (Figure 5) shows that when the surface temperature exceeds 110°F, then the emissions tend to rise.
Optimizing Performance
Synagro’s commercial scale R&D program demonstrated the +ASP/wBFC technology was operationally feasible, keeping in place a watered, temperature controlled ASP and producing quality finished compost. Key operational needs related to maintenance of the finish compost layer is critical to success, in combination with maintaining acceptable oxygen and temperature conditions beneath the biofilter layer.
The operational cost savings are found in eliminating the need for high horsepower negative ASP with biofilter composting strategies. Further, the low flow positive air fan energy consumption is less than that of the negative flow air fans used for negative ASP and mechanical biofilter composting technologies. With proper site operation and maintenance of the biofilter cap +ASP/wBFC shows great promise for improving composting site sustainability.
Tony Cordova and John Goodwin are with Synagro. Tom Card is an Emission Estimation Expert and C.E. Schmidt is an Emission Assessment Scientist.