Composts and organics should be emphasized as much as efficient irrigation techniques to keep crops growing in these times of uncertain water supplies. Part I
Sally Brown
BioCycle March/April 2014
No amount of conservation and soil improvement can make it feasible to live or farm in a world without water. When conditions are as bad as they have been in California this winter, with less than 20 percent of typical snowpack, it is near impossible to prescribe steps to ward off a crisis. With that said, there are many things that can be done to reduce the severity of the crisis. In addition, for years with below normal rather than no snow pack, it is possible to improve water efficiencies so that only minimal impacts are felt.
By water efficiencies, I am talking about altering our management of organic and water residuals to make optimal use of our water resources. With fluctuations in rainfall and water availability predicted to increase rather than stabilize, it is important to understand what can be done and to start doing it — whatever the snowpack or forecast. This article focuses on how residuals can provide a critical tool to lessen the impact of both above and below normal precipitation.
Urban dwellers typically do not realize that a majority of water resources are used for crop irrigation rather than domestic uses. In California, over 75 percent of the potable water resources are used to grow food. The amount of water needed to produce a crop will vary based on the type of crop, the irrigation systems and the climate.
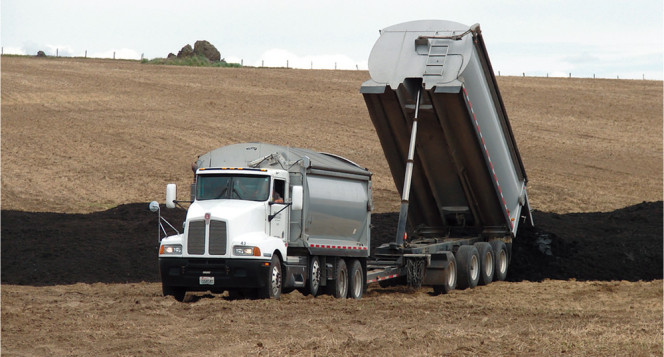
A long-term study testing biosolids as a fertilizer for dry land wheat in Washington State evaluated the connection between soil organic matter and water retention and infiltration.
Some Basic Facts
Plants need water to grow. In the reaction that transforms atmospheric carbon dioxide to plant matter, water and carbon dioxide are the key required ingredients to create starches, sugars and proteins — all the good things that come from photosynthesis. Climate can impact water demand above what is required for photosynthesis by increasing transpiration demand. Transpiration is the process of water movement from plant roots up through leaves and into the atmosphere. High temperatures, low humidity and blue skies lead to high transpiration demand. Plants have to maintain equilibrium in internal water pressure, and similar to how people sweat, high temperatures and low humidity will force plants to release water into the atmosphere.
Different plants have different transpiration demands and efficiencies. If there is too high a transpiration demand and too little water entering plant roots from soils, different plants will react differently. Lettuce, which is almost all water, will wilt very quickly if temperatures get too hot and transpiration rates are too high. Corn leaves will curl as a way to reduce the transpiration demand, much the same way that people hide under a blanket to avoid sunburn at the beach. Lawns are like lettuce — they need a tremendous amount of water. Native plants, in regions where water is scarce, will need much less water. Hence the emphasis on removing or burying lawns and replacing them with native plants in places like Petaluma, California (see “Teamwork,” February 2012).
Plants get their water for photosynthesis and for transpiration primarily from the soil. Water stored in soils, often termed “green water,” is a significant component of the hydrologic cycle. Water enters the soil through rainfall or irrigation. This is not a 100 percent efficient process. If the rainfall or irrigation comes too quickly, the soil won’t be able to soak up the water fast enough, and a portion of the water will flow off the soil surface, often carrying some soil with it. If the water is able to flow into the soil, a portion of it will stay in the surface near the plant roots. Excess will flow through the soil to rivers or to groundwater. The goal is to have as much water enter into the soil and stay where plants can access it. This will let the plants grow, stop the lettuce from wilting and uncurl the corn.
Soil properties control how efficiently soil and water interact. Heavy or high clay soils are slow to let water infiltrate but will hold onto it tightly, preventing or slowing flow to groundwater. Clay particles are tiny, too small to be seen without a microscope or felt by fingers (< 0.002 mm). Light soils or high sand soils will let water infiltrate rapidly but have a low water storage capacity. Sand particles are gigantic — big enough to be seen by the naked eye and felt by your fingers (0.02-2 mm). Silty soils, in between sand and clay, are an ideal texture for water infiltration and storage (0.002-0.02 mm). The particles are coarse enough to allow for infiltration and fine enough to store a large portion of the water. However, these soils are prone to erosion, because the particle sizes are just big enough that they don’t stick together well, but light enough to be carried by water and wind.
Enter Organics
How do organics impact the interactions between water and soils?
It is near impossible to change the texture of your soil. The top six inches of soil typically weigh about 1,000 tons/acre, with the vast majority of that weight associated with the sand, silt and clay particles. If you packed sand, silt and clay together really tightly (i.e. pressed them back into rocks), they would weigh 2.65 grams per cubic centimeter or 2,000 tons/acre. That is almost double the weight of the same amount of soil. The difference between what a 6-inch thick, acre wide slab of rock weighs and what the soil weighs is due to pore space and organic matter. Soils typically consist of between 0.5 to 5 percent organic matter by weight, and 30 to 60 percent pore space by volume.
Increasing the organic matter concentration of soils increases the amount of pore space by providing “glue” that can aggregate the mineral particles. Together, these aggregates create a network of pore space in the soil. This increased pore space allows water to flow into soils more quickly and provides surfaces that can hold onto the water once it is in the soil. The aggregates also hold the soil particles together. This aggregation makes them more resistant to erosion. The most effective way to increase a soil’s organic matter content in a short period of time is to add organic amendments to the soil. Composts, biosolids and manures will all do the trick. How much difference organic matter can make will vary based on a number of factors. However, it will always help and it can help a lot (Table 1).
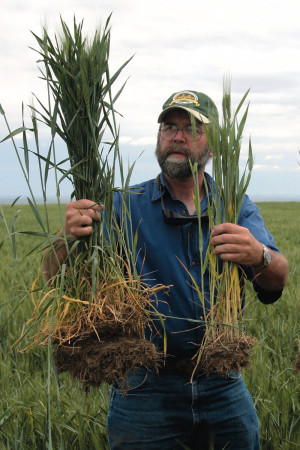
The wheat yield increase across all harvests was 16 percent due to higher water holding capacity.
(Image courtesy of King County Wastewater)
A long-term study testing biosolids as a fertilizer for dry land wheat in Washington State evaluated the connection between soil organic matter and water retention and infiltration (Brown et al., 2011). The soil was classified as a loamy sand — considered a near ideal texture (15% clay, 45% sand and 40% silt). The bulk density was low (1.3 Mg m3), which is a sign of a good soil. Wheat is planted in these soils every other year, with a year of fallow to conserve moisture. Average rainfall is about 10.6-inches/year. In years of close to average rainfall you get wheat. When the rainfall is at a certain point below average you get some straw.
Biosolids had been applied every four years at cumulate application rates of 18 to 40 Mg/ha. By adding the biosolids, the soil organic matter was increased from 1.5 to 3 percent. This resulted in increased water holding capacity at the higher loading rates of about 10 percent. Moisture is what limits yield at this site. Conventional fertilizers provided nitrogen but did not change soil carbon therefore no impact on soil moisture was observed. A model developed by Washington State University was used to predict the yield increase from this increased water holding capacity. The model predicted increases of 10 to 20 percent. In reality, what happened was yield increases across all harvests of 16 percent. For the last two out of three harvests, these increases were greater than 25 percent. Average yield for wheat in Washington is 67.3 bushels per acre. The 16 percent increase means an average yield of 78 bushels per acre. Using an average price for wheat, that is net revenue of $593/acre compared with $512.
There are limits here to how much organics can do. In 2002, the two-year moisture cycle for the wheat fallow rotation was less than 70 percent of normal. The biosolids crop yields and yields of all other treatments were low; food can’t be grown without water. To apply these research findings to agriculture in California, we can look at hay, a grass crop that is similar wheat. Sales of hay in California were about $1.4 billion in 2012. If biosolids were used to fertilize the hay instead of synthetic nitrogen, and if comparable yield increases were observed as a result of increased water availability, additional revenue of over $100 million would be realized.
Application To Tree Fruit
The same study in Washington State also looked at changes in soil moisture following compost application to tree fruit. Tree fruit is a big industry in both Washington and California. In fact, California is the largest producer of almonds worldwide. For this site, the soil texture was a silt loam, and after the farmer had applied about 40 tons/acre of compost made from food processing wastes over several years, the soil carbon increased by about 24 percent. Bulk density also decreased from 1.2 to 0.9 Mg m3. We measured changes in soil water holding capacity and saw an increase of about 50 percent in the soils that had gotten compost. The field sampled was planted to cherry trees. These trees use about 3.5 acre-feet (the amount of water required to reach a depth of 1 foot for 1 acre) each season. An acre-foot is equivalent to 325,850 gallons. Compost reduced the water required by close to one acre-foot per acre.
According to a University of California Drought Management Fact Sheet on Almonds (Division of Agriculture and Natural Resources, 2014), almond trees in California use between 41-inches and 54-inches/year of water or about 3.5 to 4.5 acre-feet. That is about the same as cherries. The same fact sheet also talks about how to keep trees alive with minimal water and how to irrigate efficiently. There is no mention of compost use. Almonds were the third biggest crop in California in 2012, bringing in close to $4.4 billion. Almonds were grown on 870,000 acres. Assuming that there was enough compost to go around (see sidebar) and that water savings were similar to what was observed for the cherries in Washington, we can figure out how much water would be saved by using compost on the almond trees. Using the numbers from the cherries, applying compost on almonds in California would save close to 870,000 acre-feet or over 280 billion gallons of water each year.
The water savings numbers are from my own research. Other studies have found similar results. Two reports by the Recycled Organics Unit of the University of New South Wales are relevant. In both studies, conclusions are based on summaries of the literature. In a report on organics use in fruit and orchard production, the authors noted increases in soil moisture ranging from 10 to 80 percent with composts applied as mulches. Yield increases of 20 to 400 percent were also observed when composts were used, but that was not in relation to water — likely just soil quality. The authors note that this does not translate directly to increased plant available water. They recommend that studies be done to quantify precise water savings. In a second report they also note the benefits for organics use in agriculture. Organics alter soil structure to increase infiltration and water retention. This effect is decreased runoff and erosion, allowing for reduced frequency and intensity of irrigation and “prolonged cropping” in dry land cultivation.
They also note the benefits of applying compost as mulch to the soil surface. Water can evaporate directly from the soil surface. Dark soil, exposed directly to the sunlight, will heat up quickly and water will be lost to the atmosphere. Mulch is a version of a beach blanket, shielding the soil from the sun. When composts are applied as mulches, studies show decreased irrigation water demand as a result of decreased evaporation from soil surfaces. Reductions in irrigation demand ranging from 30 to 70 percent have been reported with the use of mulch (ROU, 2007).
Studies on the benefit of compost to water retention have been conducted by California researchers as well. A report by the California Air Resources Board and California EPA, designed to quantify greenhouse gas emissions associated with the production and use of compost, also evaluated properties of compost, including increasing water retention properties of soil (CARB-CalEPA, 2011). Compost use and water savings were quantified in terms of decreased water use and decreased soil erosion. Based on two California studies, the report found savings of 185 gallons of water per ton of compost used to restore a burn site, and 678 gallons of water per ton of compost at a construction site for the first year after compost was applied. Considering decay of compost, they extrapolated these numbers to result in 3,550 and 13,000 gallons of water savings for each ton of compost applied to the burn site and construction site, respectively, over a 30-year time frame. They found annual soil erosion savings of 91 and 328 lbs per ton of compost used for fire and construction respectively. This was calculated to be equivalent to 1,750 and 6,300 lbs of soil per ton of compost respectively over the 30-year time frame.
Use of organics in agriculture is likely to generate significant water savings through multiple mechanisms. More water will soak into soils, less water will flow over soils, and soil erosion will be reduced. Increased soil water will result in greater drought tolerance for non-irrigated crops and less frequent irrigation for irrigated crops. Organic mulches will reduce evaporation of water from soils, also reducing irrigation demands. While the precise amount of water that is saved will vary based on specific soil, plant and climate factors, there is no doubt that water will be saved. It is time for water benefits associated with organics use to be recognized. Composts and organics should be emphasized as much as efficient irrigation techniques as a way to keep growing in these times of uncertain water supplies.
Sally Brown is a Research Associate Professor at the University of Washington in Seattle and a member of BioCycle’s Editorial Board. Part II of this article series examines the role of recycled organics in urban watersheds.