Top: Digestate application. Photo courtesy of John Rennie & Sons and Bryan Lewens.
Sally Brown
Methane has been big on the global stage recently. At the climate meeting in Glasgow, Scotland last fall, upwards of 30 nations signed a pledge to reduce methane (CH4) emissions ASAP. This makes sense. A short life span in the atmosphere coupled with options in hand to reduce emissions (get that food waste out of the landfill) make reducing CH4 a no brainer in terms of global priorities. Diverting food scraps is gaining traction and new possibilities.
With SB 1383 in California and new programs to reduce food waste in general, it almost seems like we are turning the tide. If we could only stop eating beef and dairy — this would truly be a guilt-free victory. While researchers work on finding the perfect Gas-X tablet for cattle, a different gas is keeping me up at night.
Nitrous Oxide 101
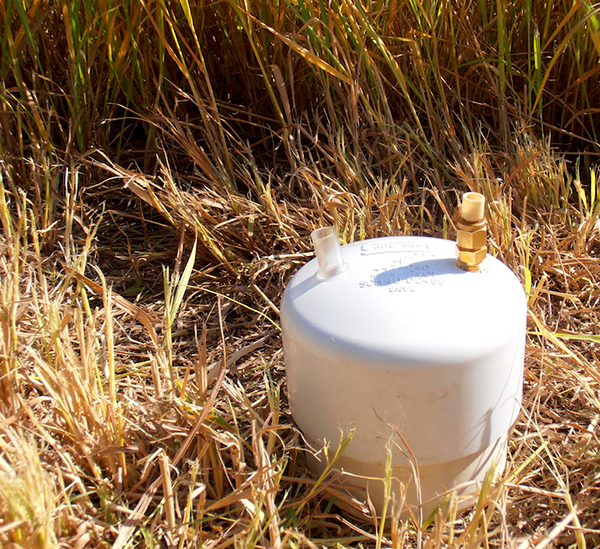
Gas chamber measures fluxes of fugitive gasses including methane and nitrous oxide. Photo courtesy Sally Brown
Nitrous oxide (N2O) is the other fugitive gas that can be formed from organics as they decompose. Unlike methane, it’s not so easy to predict exactly when it will form or what to do to prevent it. If you think that methane is powerful, N2O is a knockout — at about 300 times the global warming potential of methane. While N2O is often referred to as laughing gas, to me it is no laughing matter. I think of it this way, at least in terms of flatulence. Methane is your normal fart. Nitrous oxide is the type of fart, referred to in my family by the code name SBD (silent but deadly), that sends everyone, including the person at the point of origin, running from the room and opening all of the windows.
The primary source of N2O in the atmosphere is from nitrogen fertilizers. The development of synthetic fertilizers — pulling soil nitrogen literally out of the air — took over agriculture starting about the mid 1900s. Engineers in Germany had figured out how to convert gaseous nitrogen into ammonia. This was an explosive finding, literally. The primary reason for this was to create nitrogen-based explosives like TNT. Research showed that it also worked to feed plants. Use of synthetic nitrogen fertilizers was part of the Green Revolution, leading to increased yields and reduced hunger worldwide.
It also greatly increased the amount of nitrogen cycling from the atmosphere into our soils and waters. As it cycles, the vast majority of that N goes back to the atmosphere as nitrogen gas. However, a fraction returns to the atmosphere as N2O. The International Panel on Climate Change (IPCC) has set a default value of 1% of all of the nitrogen fertilizer that is used as the fraction that becomes N2O. If you take that 1% and add it to the energy required to make the fertilizer in the first place you can see why reducing dependence on synthetic fertilizers is a good deal:
- Each kg of N as ammonia requires the equivalent of 4 kg of CO2 to produce
- 1% of 1 kg = 10 grams of that fertilizer N goes back to the atmosphere as N2O
- 296 (CO2e of N2O) x 10 g = 2960 g or 2.96 kg of CO2e (CO2e is carbon dioxide equivalent, a greenhouse gas measurement)
- Total emissions associated with 1 kg of N fertilizer = 4+2.96 or 7 kg CO2
That is a very high number. My first thought when I see that number is that this is even more reason to recycle every bit of organics back to soil. Each kg of N in the compost that goes back to the farm is one less kg of ammonia that needs to be manufactured, sort of. In reality not all of the nitrogen in those organics is ready to be used by plants. In fact, if you want one kg of N for a plant, you typically need to add at least twice that much total N if you are relying on compost, biosolids or manures to meet your nitrogen need.
Organic Nitrogen 101
When nitrogen is added to soil in composts, biosolids and manures, it is typically added as organic nitrogen. An easy way to think of this is the protein component of steak. All living things need nitrogen and so all living things contain nitrogen. While you and I might like a ribeye, plants enjoy their nitrogen in a mineral form. When the microbes chow down on soil organic matter, they convert the organic matter that they don’t need into ammonia. Another set of microbes will convert that ammonia into nitrate. They do this because it tastes delicious (to them) and because it releases energy. This nitrate is the preferred flavor of nitrogen for most plants. Any excess is converted back to nitrogen gas. This whole process is referred to as the nitrogen cycle. Take a soils class or an environmental studies class and I promise you will learn about the nitrogen cycle. It is basically an example of microbes in action, transforming nitrogen to release energy or as a place to stuff leftover electrons from eating carbon. Let me focus on that last bit.
When nitrogen is present as nitrate it has the chemical formula of NO3-. That translates to one nitrogen hanging around three oxygens with the whole group having a charge of -1. Each of those oxygens will have two negative charges for a total of -6. The fact that the grouping has a charge of -1 tells you that the N has a charge of +5. As a gas that nitrogen has a charge of zero. Microbes turn the N in the nitrate back to nitrogen gas. They do this by shoving 5 electrons onto it. Those come from eating carbon compounds. The electrons have to go somewhere and if there is not enough oxygen around (where us aerobic eaters put electrons, turning oxygen gas into water), nitrogen in the form of nitrate is a pretty good substitute. This is what happens in wetlands and why they are so essential for water quality. The microbes in the wetlands take any nitrate dissolved in the water and send it back to the air as nitrogen gas.
Not Always Hunky Dory
Here is the catch. The reaction doesn’t always go as planned. When it gets interrupted or only partially completed you get nitrous oxide. We can count the charges on N2O. The O will be -2 and the whole molecule has no net charge so that means that each N is a +1. So close to nitrogen gas but just not close enough. The conditions that you need to form N2O are low oxygen, available NO3-, and good carbon to eat. At first, I thought that composts and other types of EOM (exogenous organic matter) would clearly be better than nitrogen fertilizers with much lower potential to form N2O. As slow-release sources of N, these materials would never flood the soil with enough nitrate to really make N2O emissions a concern. Then I realized that while they don’t flood soil with NO3-, they do provide lots of tasty morsels of carbon for the microbes to eat. In addition, because application rates to meet the nitrogen needs of the crop mean double or more the total nitrogen added with fertilizers, there is a potential for N2O emissions from organics to be a real concern — aka the stuff that keeps you up at night.
Are my nightmares based in data? My go to expert for research on N2O is Philippe Rochette, a scientist with AgCanada. He has done some excellent research on this gas and identified conditions where it is likely to form. Luckily for the world, it is not a regular feature on agricultural fields. As I said earlier in this column, it is the exception, not the rule. Nitrous oxide typically occurs in spikes; none, none, none and then A LOT. It is typically formed in finer textured soils (higher in clay) after significant rainfall or with the spring thaw.
In recent review papers, Rochette and his coauthors have refined the default factors used by IPCC to be more reflective of specific conditions. In Eastern Canada where it rains, N2O emissions factors (from a survey of the literature) for synthetic fertilizers ranged from 0.45% for medium textured soils to 3% for fine textured soils. Emissions factors mean the percentage of total nitrogen that is estimated to be released as N2O, so when you get up to 3% of total N and you factor in the CO2 equivalence, that really adds up. In other words, from half of the default value to 3x the default value. The same paper included estimates for organic sources of N. Those ranged from 0.28% to 2.76% for coarse and fine textured soils. While lower than from synthetic sources, remember that you typically apply at least twice as much. That packs double the punch. They also found that emissions in Western Canada were pretty much negligible, things to daydream about rather than to have nightmares over. Emissions from annual crops were much higher than for perennials: 2.1% versus 0.4%.
In a separate paper, Rochette and his coauthors also refined default factors for different types of organic amendments. These are ranked high, medium and low risk. High risk materials include animal slurries, wastewater and biosolids (1.21% emission factor). The medium risk includes solid manures (0.35%) and the low-risk group (0.02%) includes (breathe a sigh of relief) composts, pelletized materials and paper mill sludges. An important caveat here is that the term biosolids is used very loosely and not likely in reference to the anaerobically digested 20%+ solids material that comes from municipal wastewater treatment plants.
Two studies on those actual materials generally bear out what these review papers suggest. In one of our studies, we used dried biosolids on a sandy soil in a dry region to grow switchgrass. Water came from irrigation pipes. We tested for N2O and found it in very small quantities. We even doubled our biosolids application rate in an attempt to find it. When we applied biosolids at the high rate, the default N2O emissions ranged from 17-27 kg N2O per hectare/year. The emissions that we observed were 0.52 and 0.37 kg N2O per hectare/year. Sweet dreams when you surface apply dry material to sandy soils in an arid climate. Another study tested biosolids and biosolids compost applied to a fine textured soil in the rainy part of Canada. The incorporated biosolids gave off a lot of N2O — an emissions factor of 1.9%. In comparison, the urea emissions were lower than the default, coming in at 0.37%. Composting made a big difference, lowering emissions to 0.19% of total N applied. The elevated emissions factor for the biosolids translates to much higher N2O for the biosolids (about 2 tons of CO2e from 193 total kg N applied) than the urea (about 0.47 tons of CO2e from 96 total kg N applied).
The authors of the paper did a partial GHG budget giving a debit to the urea for cost of manufacturing and not giving any credits to the biosolids for avoiding that cost. Their totals came to 1 ton of CO2 per hectare for the fertilizer and 2.1 tons for the biosolids. Because using the biosolids also saved the CO2 from fertilizer production and got additional points for phosphorus and soil carbon, the pain is somewhat lessened …. but still. This is too close for comfort in my book. The really good news here is how low the emissions were from the biosolids compost.
Good New For Biosolids Compost
The take home here is that composts can add reduced N2O emissions to the long list of benefits in comparison to synthetic fertilizers. That is the sweet dreams part of the news. For biosolids and for other nitrogen rich materials coming out of digesters, whether those are mechanically operated or in animal bellies, the take home is that N2O is something to take very seriously. High emissions can wipe out the rest of the carbon benefits that come from using these materials. If you are applying to fine textured soils in parts of the world where rain does not come from an irrigation pipe, it might make sense to at least partially compost your materials before you land apply. For restoration sites where higher than agronomic rates of material are used, that becomes a definite.
To date there is limited research on N2O emissions associated with the types of materials that we have ready access to including food/yard trimmings composts and municipal biosolids. The little information that is out there suggests more research is needed. More tools need to be identified to reduce the potential for the GHG equivalent of SBDs to be commonplace. In the meantime, chances are good that I’ll keep having those nightmares.
Sally Brown, BioCycle Senior Adviser and Connections columnist, is a Research Professor in the College of the Environment at the University of Washington.