Field-scale trial utilizes urban soil husbandry practices — compost amendment and soil decompaction — to earn an impervious area reduction credit.
Craig Coker and Stuart Schwartz
BioCycle March/April 2017
Storm water runoff management in the U.S. has been shifting from solely water quantity to both water quantity and quality management for the past decade. This refocus has made many storm water management professionals aware of the inherent values of compost-amended soils in terms of runoff reduction from improved infiltration, improved soil water holding capacity and improved soil ecologic health. This combination of benefits is often grouped under the term soil husbandry.
Due to water quality constraints in many streams, embayments and estuaries, storm water runoff is increasingly regulated and subject to discharge permit limits. The Chesapeake Bay in Maryland and Virginia is an example of an embayment with water quality problems. The watershed of the Chesapeake Bay spans 7 states draining almost 64,300 square miles (about 2.1% of the land area of the contiguous 48 states in the U.S.). The U.S. Environmental Protection Agency (EPA) has imposed Total Maximum Daily Loads (TMDL) for nitrogen, phosphorus and sediment entering the Bay from point sources like water resource recovery facilities and nonpoint sources like storm water runoff from cities and industries and agricultural runoff from farms. The TMDLs are apportioned to the seven watershed states in the form of waste load allocations and it is up to each state to reapportion those allocations to the various sources of nutrients entering rivers feeding the Bay in their states.
Storm water runoff from cities and towns is often regulated through municipal separate storm sewer system discharge permits issued by the states (MS4 permits for short) under the National Pollutant Discharge Elimination System (NPDES) program administered by EPA. In Maryland, the MS4 permits establish two specific requirements for developing restoration plans. The first involves restoration of 20 percent of a jurisdiction’s impervious surface area that has little or no storm water management. The impervious area restoration requirement is part of the strategy in Maryland’s Watershed Implementation Plan (WIP) for meeting the Chesapeake Bay TMDL. The second requirement is to develop a schedule for Best Management Practice (BMP) implementation to meet all applicable wasteload allocations (WLAs).
The restoration requirement is based on the demonstrated improvement to runoff water quality of having permeable (or pervious) areas that allow infiltration of rainfall into the soil compared to impervious areas where rainfall runoff is captured, controlled and discharged. Restoration of impermeable areas to pervious conditions reduces runoff and the nutrients lost in storm water runoff, thus entitling the host jurisdiction to nutrient “credits” towards meeting their assigned storm water waste load allocations. Credits are also earned by retrofitting water quantity management storm water systems to have a water quality improvement feature (e.g. converting a dry detention pond to a wet retention basin).
Restoring Pervious Surfaces
A key consideration in planning storm water retrofits and new storm water management systems in Maryland is a concept known as the Water Quality Volume (denoted as the WQv), which is the storage needed to capture and treat the runoff from 90 percent of the average annual rainfall. In numerical terms, it is equivalent to an inch of rainfall multiplied by a volumetric runoff coefficient and the site area. The volumetric runoff coefficient, Rv, in turn, is most commonly calculated as a function only of percent impervious land use cover (i.e. that fraction of a drainage area covered with impervious surfaces like asphalt and concrete). This has led many storm water management professionals to concentrate only on pervious surfaces in new construction (porous pavement, etc.) and on restoration activities that reduce impervious cover.
However, the reliance on new or restored pervious surfaces misses a key consideration in BMP design — not all pervious surfaces are equal in their infiltration capacities. The greenspaces in constructed environments (lawns, parks, golf courses, etc.) often have much higher runoff characteristics and lower infiltration characteristics than native forest floor soils. Storm water runoff calculations are based, in part, on the Hydrologic Soil Group (HSG). Soils are classified by the USDA’s Natural Resource Conservation Service into four HSGs based on the soil’s runoff potential. The four HSGs are A, B, C and D, where A’s generally have the smallest runoff potential and D’s the greatest. HSG designations are routinely derived from county soil maps and rarely account for the inherent disruption and compaction of urban soils that routinely accompanies mass grading and site preparation.
It was the recognition of the unequal nature of runoff from pervious areas that led, in part, to the development of the Soils for Salmon program in King County, Washington in the late 1990s, which institutionalized soil husbandry practices for onsite management of storm water runoff. Increasingly, soil husbandry practices are being recognized as essential tools to restore environmentally sustainable features in human-altered landscapes. Yet, an unanswered question persists: How to prove that urban soil husbandry, with all its associated benefits, will remain viable, and the benefits will persist, on time scales commensurate with structural storm water management alternatives (i.e. 10 years or more).
Soil Decompaction Trial
The Center for Urban Environmental Research and Education at the University of Maryland, Baltimore County partnered with the Baltimore Department of Public Works to evaluate this question through a field-scale implementation of soil decompaction and compost amendment. An asphalt parking lot was converted to a turfgrass field at an elementary school in Baltimore, Maryland in 2010. This conversion was intended for Baltimore to earn an impervious area reduction credit under the City’s MS4 permit. This process of soil decompaction and compost amendment is termed suburban subsoiling, drawing upon the established agricultural practice of subsoiling and applying it to the urban landscape.
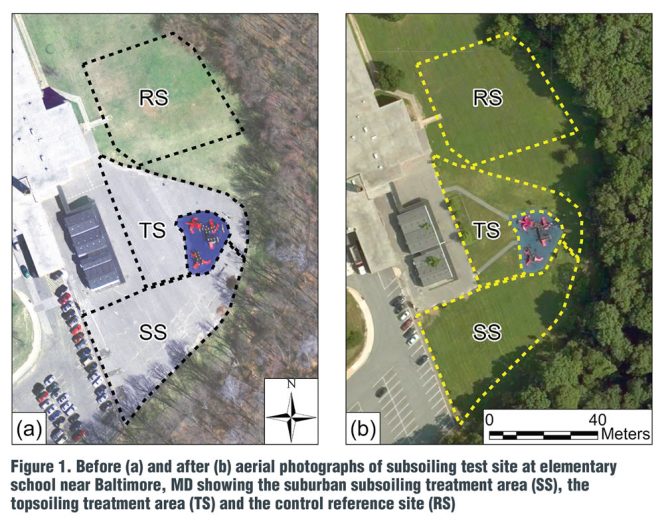
Figure 1. Before (a) and after (b) aerial photographs of subsoiling test site at elementary school near Baltimore, MD showing the suburban subsoiling treatment area (SS), the topsoiling treatment area (TS) and the control reference site (RS)
The SS treatment consisted of deep-ripping the study area to a depth of 20 inches (50 cm) and surface-spreading a 3-inch (7.5 cm) average depth layer of a very stable and mature leaf compost (Leafgro® compost from Maryland Environmental Service) containing 1.9 percent total nitrogen. The compost was incorporated to a 5-inch depth (12.5 cm) using a chisel plow targeting an estimated 2:1 soil-to-compost ratio. The TS treatment consisted of spreading 2 to 3 inches (5–7.5 cm) of topsoil over the soil exposed by removal of the asphalt parking lot and base. In August 2010, both the SS and TS fields were hydroseeded with a tall fescue-bluegrass-rye grass mix. No supplemental chemical fertilization or irrigation has been conducted on either site, just routine grass mowing with clippings being recycled in place.
Soil from all three test areas was sampled and characterized seasonally from 2011 to 2013. These data are summarized in Table 1.
In addition, in-situ soil strength was measured with a GPS-referenced cone penetrometer. Cone penetrometers measure relative soil strength by measuring the pressure needed to push a cone-tipped rod into the soil at a constant measured rate. The instrument measures a cone index, in megapascals (MPa) and, in agriculture, a cone index greater than 2.1 MPa is considered to impede the root growth of most plants, while values less than 1.4 MPa are considered to offer low physical resistance to root penetration. When measured almost two years after treatment (July 2012), the cone index for the TS area was greater than 2.1 MPa at a depth of 4 inches (10 cm), suggesting significant shallow rooting resistance while the cone index values for both the RS and SS areas remained less than 2.1 MPa to a depth of 12 inches (30 cm) in both fields.
Soil moisture was measured by inserting a soil probe into the upper 3 inches (7.5 cm) of the soil profile. As noted in Table 1, soil moisture was consistently higher in the SS area (35.3%) than in the TS or RS areas (25.6% and 23.4% respectively). This suggests that water holding capacity in the root zone is compromised by standard grading and topsoiling measures. The significantly higher soil moisture in the SS field supported very vigorous and drought-resistant turf, which was absent in the TS field. This high mean moisture content was also associated with significantly higher sustained levels of soil organic matter.
The hydrologic benefits from suburban subsoiling were estimated to simulate design storm runoff using computer modeling of ten 24-hour storms of varying recurrence intervals. Storm event precipitation and simulated runoff were used to estimate an effective runoff curve number (ECN, a dimensionless parameter used in estimating runoff from various land uses) and an expected Runoff Coefficient, Rv. The expected Rv values for the three fields (Table 2) suggest that suburban subsoiling could reduce the runoff from the TS field by a factor of 30. The ECN estimates were derived from finding the curve number that best fit the simulated rainfall-runoff response from all 10 design storm events.
Quantifying the hydrologic impact of soil decompaction and compost amendment with a site-specific ECN or Rv provides storm water design professionals a consistent means to integrate suburban subsoiling with current practices in site development and storm water management design, along with a hydrologically rational basis to consider runoff reduction credits, storm water nutrient trading, and efforts to establish performance criteria for the design of sustainable landscapes.
Craig Coker is a Senior Editor at BioCycle. Stuart Schwartz, Ph.D. is a Senior Research Scientist with the Center for Urban Environmental Research and Education at the University of Maryland Baltimore County. He can be reached at stu_schwartz@umbc.edu. Figure and tables in this article are reprinted from Journal of Hydrology, December, 2016, Stuart S. Schwartz, Brennan Smith, Restoring hydrologic function in urban landscapes with suburban subsoiling, Pages 770-781, Copyright 2016, with permission from Elsevier. This article is based on the referenced peer-reviewed scientific journal article.
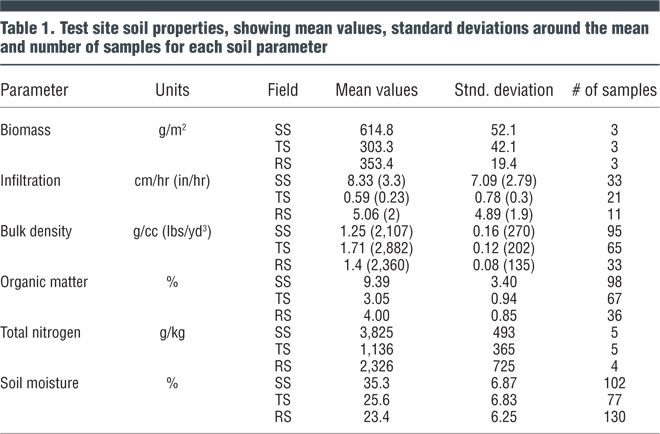
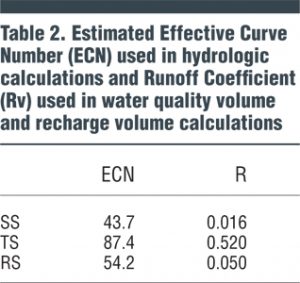