Top: The compost-amended Phase 3 Bioswale under the Aurora Bridge in Seattle can treat 1.9 million gallons of storm water annually. Photo by Site Story
Sally Brown
Compost and urban soils are a match made in heaven. Composts give urban soil the organic matter it needs to help plants grow. It soaks in storm water, making those same urban soils more drought resistant. With compost, lawns are watered once a week instead of once a day. Forget those ChemLawn (now TruGreen) trucks. The compost will also keep lawns green and lush.
What is not to love? That is what the compost producers say. Too often municipalities won’t swipe right. Because in addition to all the wonderful things about composts, they always contain phosphorus. Instead of seeing the rainbow world of possibilities that compost can provide, many cities, concerned about eutrophication and water quality, only see things in black and white: no phosphorus or not allowed. Here are some tools to help bring that compost color into regulatory acceptance.
Nitrogen And Phosphorus 101
A primary concern regarding nitrogen (N) and phosphorus (P) is an excess of both can cause eutrophication of lakes and ponds. Any organic (plant or poop based) soil amendment will contain both as both are necessary nutrients. In fertilizer form, it is possible to add just N or just P. In places where regulators are concerned about P, lakes and ponds are likely P limited. That means that P is the element that is in short enough supply that adding more will cause eutrophication. Adding additional N in those systems won’t cause the algae to grow as they already have enough N.
The first thing to communicate is that while phosphorus always shows up with nitrogen, the two are nothing alike. Nitrogen is all loose and wild. It can go up, down and sideways. To put that in less risqué language, nitrogen can move through soils into water (down), it can turn back into nitrogen gas when soils get saturated (up) and it can move on soil particles during rain and wind storms where soils erode (sideways). Phosphorus only moves in one direction — sideways. Unlike nitrogen, phosphorus is not soluble in soils so moving down is not an option. It only moves when the soil it is stuck on moves. This give you the first example to show why compost should be courted and not ghosted.
Compost helps soil form aggregates or stable clumps of soil. When soil is well structured or well aggregated its bulk density (how heavy it is) goes down. That gives water more room to move in and move in more quickly. The official terms for that are increased infiltration rate and increased water storage capacity. If the water is soaking into the soil rather than carrying the soil with it as it goes into storm sewers, that means that less phosphorus is moving. It is one thing to say that. It’s another to show that with some numbers.
P Math
Let’s start with soil without compost and a storm comes. We can say that each liter of water that erodes off a soil during a storm event carries 10 grams (g) of soil and each g of soil carries 10 milligrams (mg) of P. If one inch (2.54 cm) of rain falls on 1 square meter (m2) and half of that erodes, the following happens:
- 1 m2 * 0.0254 m (depth of rain) * 1,000 liters per m3 of water = 25.4 liters
- 25 liters per m2/2= 12.7 liters of storm water
- 10 mg P per g soil * 10 g soil per liter * 12.7 liters of storm water that erodes = 1,270 mg of P
A quick search tells me that an average city block is 200 m long. If you assume that the grass parking strip between the sidewalk and the curb on that block is 1 m wide you have 200 m2 for each block. That means that each city block during that same rainstorm will end up losing the following:
- 1,270 mg P per m2 * 200 m2 per city block = 25,400 mg P or 254 g of P. That is about half of a pound. Keep that up for a few blocks and before you know it you are talking kilograms of P lost with a mediocre storm event.
But what happens if you add compost? Even if that compost contains P it will still stop the vast majority of that storm water from running into the street and carrying soil with it. That is why compost is the main ingredient in rain garden and bioretention soils. Even if the compost doubled the concentration of P in the soil (never going to happen but makes for a good example) you still come out ahead. Here’s the math.
If that same 1 m2 of soil had compost on it and got that same 0.025 m of rainfall, it is likely that instead of 12.5 liters running off the surface, 2 liters would run off. In addition to less water running off, that water would likely be carrying less soil, but here we can assume that the soil load is the same:
- 10 g of soil per liter with 20 mg (double the previous amount) of P per g * 2 liters of water = 400 mg of P
- 400 mg P per m2 * 200 m2 per city block = 80,000 mg P or 80 g of P. That is a very conservative estimate and also much less than 254 g of P.
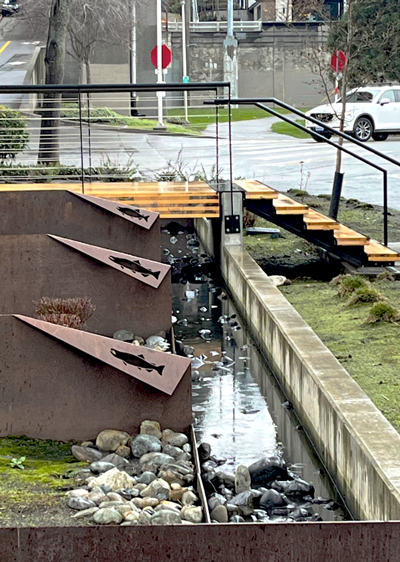
Treated water from the bioswale after record rainfalls in Seattle in December 2023. Photo by Site Story
The much more likely scenario with compost use is that both less soil and less water will leave the soil and that the soil that does leave the surface will not have appreciably more P than before the compost was applied. That is why in the Pacific Northwest we have Soils for Salmon. This program is based on reducing storm water inputs to freshwater by improving soils. It finds the best way to do this is to add compost. Another example is Salmon-Safe in Seattle (WA), which targets treatment of runoff from bridges crossing Seattle’s Lake Washington Ship Canal. The untreated runoff degrades the water quality, negatively impacting migrating salmon and resident orcas. The organization installed bioswales amended with compost donated by Cedar Grove to treat the runoff before it is discharged into the canal. The project was managed for Salmon-Safe by Site Story.
Compost Binds Phosphorus
The other thing to talk about is how compost can bind P. Ron Alexander, Principal of R. Alexander Assoc., Inc., is an expert here and has written excellent articles on appropriate ways to measure available P (see references). He makes it clear that using tests to measure plant available P is not the same as tests that measure the P that has the potential to do environmental harm. Understanding this difference and why some tests are better than others will make your case stronger and more convincing.
The fact that soils can bind P is what got us into this P problem to begin with. Extension agents saw that only a small fraction of the fertilizer P applied was available to plants. To make sure that there was enough for the grass they just recommended that you add more and a little more on top of that. Many soils now measure excess available P as a result. That excess P is responsible for algal blooms and low dissolved oxygen. The regulators that don’t want you to use compost are not just out to bad mouth compost — their primary goal is to protect water quality. While I said earlier that P only moves sideways, that is not completely the case. With lower detection limits it is also possible to measure P in storm water including storm water that comes off soils. The next point is how to reduce the amount of P, however small, that comes off of soil particles into water.
The glue in soil that holds onto P are clays that contain iron and/or aluminum. These clays welcome the P with open arms and bring them right into the family. That is another way of saying that the P absorbs onto the surface structure of the oxides and effectively precipitates, making it environmentally unavailable. Ron Alexander recommends measuring water extractable P and that is an excellent measure of the quantity of P available to travel (Alexander, 2016). You can also use extracts to measure whether those iron (Fe) and aluminum (Al) oxides are full to capacity or if they have additional seats at the family table. This is referred to as phosphorus binding capacity. You compare that to the amount of P that is thinking of leaving the nest and you have a ratio. This ratio is a way to predict whether P in solution is a reason to prohibit compost or to order more.
Depending on the extract that you use to get the ratio the end result is either referred to as the Phosphorus Saturation Index (PSI) or the Phosphorus Saturation Ratio (PSR). The PSI is calculated using an ammonium oxalate extraction. The PSR is done with results from the Mehlich III (M-III) soil extraction. Both can work. The PSR is easier to come by as many soil testing labs routinely use the M-III to measure soil fertility. Just ask the lab to use that test method and include iron and aluminum in addition to P in your results.
To calculate the ratio, you just take the extractable P and divide by the sum of extractable iron and aluminum (Figure 1). A note here: the lab will give you the results in mg per kg or parts per million for each of these elements. To get the ratio, you have to convert those numbers to the number of moles of each. Moles are based on the formula weight (FW) of each element, a number that is on every periodic table. The FW for P = 31 g, Fe = 56 g and Al = 27 g. To get the moles of each you divide the test concentration by the FW. Moles are measured in grams and the test results are milligrams, so you are effectively finding the millimoles (1/1000 of a mole) of each. Here is a sample calculation:
Say that your M-III test results came out to 50 mg for P, 100 mg for Fe and 200 mg for Al
- Millimoles of P = 50/31 or 1.6
- Millimoles of Fe = 100/ 56 or 1.8
- Millimoles of Al = 200/ 27 or 7.4
To get the PSR you take the moles of P and divide by the moles of Fe + Al:
- 1.6 P/ (1.8 Fe + 7.4 Al) = 0.17
That ratio suggests that there is plenty of room at the table for added P. A ratio of <1 means that there is less P than there are places to absorb it.
Designer Composts
You can also make designer composts. By adding Fe or Al to your compost you can increase its ability to bind P, making it an effective solution rather than a potential problem (at least in a regulator’s eye). You can also, if you’d like, charge more for it. In cities that offer drinking water there are leftovers from treating that water. Typically drinking water is polished by running it through Fe or Al oxide materials. Once used, these materials are primarily landfilled. Spent water treatment residuals (WTR) still have plenty of sticking power left. We have done a lot of research looking at adding different WTRs to different composts and biosolids to increase their P binding capacity using both the PSI and PSR as indicators (Figure 2). They don’t all work equally but they all work.
While you can’t order spent WTRs from Amazon, they are typically available if you talk to the Water Department in a municipality. If you suggest that you could accept these materials for less than the cost of landfilling, you could likely get as many tons as you need. Granted, these materials are only available at certain times during the year so you would need to plan ahead. You would also have to figure out the right amount to add to bind up some but not all of the P. Once you got going on this though, you could make special blends to be used specifically to absorb extra P from storm water.
Hopefully this has given you some useful tools to convince those uneasy that compost is a cure rather than a concern when you are worried about water quality. It might even have given you some ideas for new compost products. Talk about a rainbow world of possibilities.
Sally Brown, BioCycle Senior Adviser, is a Research Professor at the University of Washington in the College of the Environment.